Research: Novel Sensors
DiSTAP will study the production of plant hormones and secondary metabolites to improve urban farming and will develop disruptive technologies for discovering plant biosynthetic pathways.
Understanding the Trafficking and Localization of Nanoparticles in Living Plants
The use of functionalized nanoparticles for targeted delivery into plant cells offers exciting opportunities in the field of agriculture, plant bioengineering and biology. However, the relationship between various physiochemical properties of nanoparticle and their ability to target different compartments within plant cells is still relatively unknown. The STRANO Lab has previously examined the subcellular uptake and cellular trapping of a wide range of polymer-nanoparticle conjugates such as various polymer wrapped SWNTs, quantum dots, and nanoceria into chloroplasts, both isolated and in living plants. Our previous work has shown that particle size and zeta potential are the key parameters that determine whether a nanoparticle will penetrate the chloroplast membrane and become localized in the organelle. Based on these observations, we developed a mathematical model of this Lipid Exchange Envelope Penetration (LEEP) mechanism that can be used to understand and engineer nanoparticles for localization in the chloroplast. We aim to identify other nanoparticle parameters to further develop predictive relationship to describe the interaction between nanoparticles and various plant membranes. The result of this study will allow us to engineer functionalized nanoparticles to be delivered specifically to certain biological compartments within plant tissues and cells for various bioengineering applications.
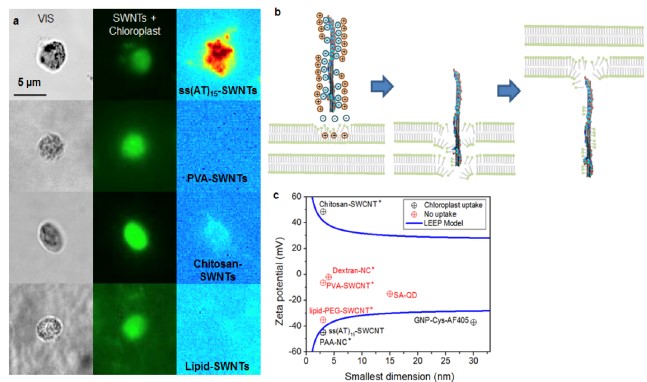
Fabrication of CoPhMoRe Sensor for Important Plant analytes
Plant hormones play a significant role in controlling plant growth and plants’ response to environmental stimuli. The development of nanosensors with high sensitivity would deepen our understanding of the complex plant signaling pathways. Our goal is to generate a library of polymers and discover an optimal CoPhMoRe (Corona Phase Molecular Recognition) sensor for specific plant hormone. Corona phase of SWCNT refers to the phase of the polymer adsorbed around its surface. The recognition takes place by the lateral interactions of the analytes of our interest with the polymer in this corona phase. Depending on the structure of amphiphilic polymers, various kinds of corona phases can be created. Using this property, SWCNT-based sensors can be developed to measure the targeting molecules within the small range of concentration. We aim to study the spatial and temporal distribution of the plants’ signaling pathways in real time and non-destructive manner with high sensitivity.
The nanosensor development will build on the Strano Lab’s existing expertise in nanoparticle localization within plants as well as through a standoff detection system that the Strano lab has developed for optical signal readout from the plant. The STRANO lab has demonstrated that living spinach plants (Spinacia oleracea) are able to function as self-powered auto-samplers and preconcentrators of nitroaromatics (pesticides analogues) within ambient groundwater. The nanosensors are embedded within the mesophyll of the plant leaf through stomatal infiltration. Thereafter, the sensors use near-infrared fluorescent light to communicate with an external receiver, and the resultant data can be sent to a user’s cell phone. As nitroaromatics in the soil are transported up the roots and stem into the leaf tissues, they come into contact with the embedded nanosensors, which report the presence of nitroaromatics in ambient groundwater through the standoff optical setup.
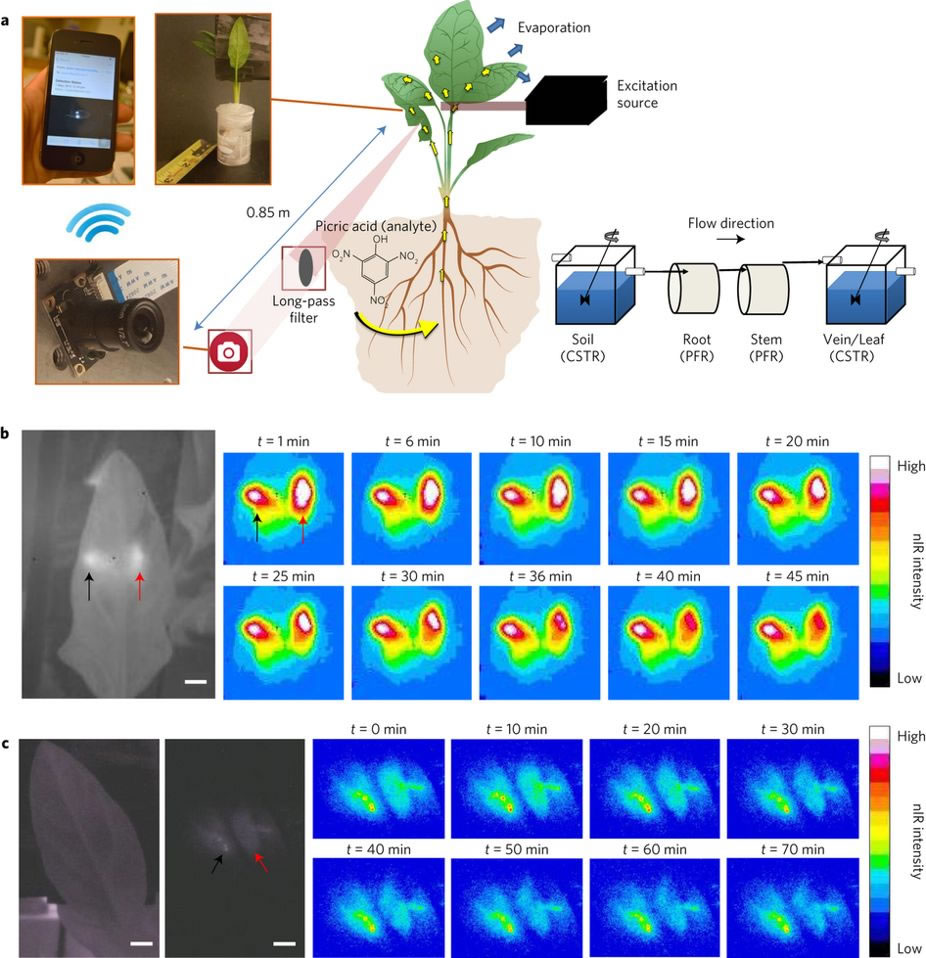
Selective Polymers for Application with Carbon Nanotubes in Sensors
Carbon nanotubes have many different chiralities, and for many applications, they may need to be sorted out into the different chiralities. For example, for use in electronic-based sensors, they will need to be first sorted into different electronic types, i.e. semiconducting ones versus the metallic ones. The CHAN lab had developed functional polymers that can selectively suspend certain electronic type (e.g. semiconducting) single walled carbon nanotubes (JACS 2013). We had also developed chromatography beads that can selectively bind metallic carbon nanotubes (ACS Nano 2016). Further, we have developed functional polymers that can richly suspend carbon nanotubes and individualize the nanotubes which is critical for them to be used as sensing components. Our polymer laboratory is well-equipped for various polymer synthetic strategies such as AROP, NCA, ATRP, RAFT, polycondensation and step-by-step peptide synthesis, and polymer characterization. We developing selective functional polymers that can finely disperse the nanotubes and that can also select plant metabolites.
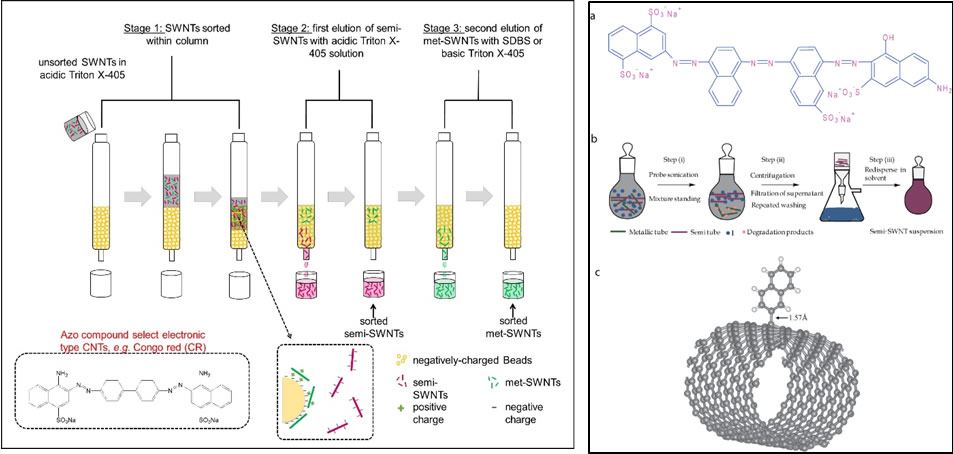
Nanosensors for Plant Drought Response
A stoma is bordered by a pair of asymmetrical guard cells. When guard cells are illuminated with light, their chloroplasts start their photosynthetic activity. This activity then translates into an intracellular osmotic pressure rise through a cascade of complex reactions, eventually leading to a stoma opening. Taking into account the stomatal geometry, we propose to design an electrical sensor as a breakable conductive wire across a stoma. Electrical contact is lost when a stoma opens and restored when the stoma closes. The stomatal aperture can hence be monitored in real time via electrical resistance measurements. Other approaches, such as stretchable strain sensors, are not applicable to stomata as they might interfere with stomatal physiology by contracting stomata during opening and by limiting stomatal gas exchange. We have formed electrodes by printing a water-based conductive ink made of carbon nanotubes and sodium dodecyl sulfate (SDS) onto the leaf. Printing patterned conductive circuits with micrometer precision across the stoma is accomplished with PDMS microfluidic chip filled with the conductive ink. Printing quality will further be evaluated by mapping leaf surface resistance using electrical probes and through confocal Raman microscopy to accurately determine the location of the carbon nanotube. Stomatal movements are tightly connected to diurnal cycles: stomata open in the morning and close in the evening. The stomatal sensor was able to detect these changes over the 7-day period as an increase (decrease) in resistance during light (dark) periods, which we attribute to stomatal opening (closing).

Determine the Impact and Toxicology of Novel Sensors on Plant Physiology
The colors we see provide us clues to the life around us. Leaves change color with the seasons. Fruits change color as they ripen and rot. Our eyes and brains observe the world through 3 color channels – red, green, and blue. These colors signal changes in the abundance of molecules – chlorophyll and carotenoids in plants. Modern spectroscopy uses 1000’s of color channels to measure and fingerprint the molecules around us. Modern computation – machine learning – allows us to interpret this riot of 1000’s of colors (the spectrum) to simultaneously monitor dozens of molecules. Advances in lasers and cameras mean that this sort of vision that can perform chemical analysis is becoming smaller and cheaper – and widely available.
The RAM lab applies spectroscopy (specifically Raman spectroscopy) to observe the inner life of plants. At SMART DiSTAP, we are exploring the application of chemical sensing to improving the productivity of farms – from outdoor fields to indoor farms. Without the ability to run, a plant relies on chemistry to rapidly respond and adapt to a changing environment and to predators. While plants appear slow-moving and essentially inert to our eyes, spectroscopy reveals the dynamic, responsive inner life of plants. Through these tools plants can ‘tell’ us when they are cold or thirsty, when there is too much light or not enough, and even if they are feeling crowded. A farmer can respond to these signals and ensure that a plant is producing optimally.